Small Mobile Instruments for Laboratory Enhancement
A student with sights set towards a career in the chemical sciences must possess a basic knowledge of chemical systems as well as have an advanced exposure to the technology used to probe these systems. Instruments are tools which chemists use to do chemistry and measure chemical processes. We firmly believe that early and repeated exposure to chemical instrumentation is the most direct and fundamental way of expressing the intrinsic connections and cooperative nature of concepts and ideas between the different chemistry courses and programs. Unfortunately, the ability of many programs and institutions to maintain and improve the quality of their educational infrastructure is compromised because of the increasing costs to maintain state-of-the-art instructional facilities and the shrinking nature of funding sources. In addition, commercial instruments are becoming increasingly more sophisticated, user interfaces are becoming simpler and more streamlined leading many of us to believe the primary educational, or professional, objective is to know how to “insert” a sample and learn the instrument software. Instruments have very much become “black boxes” and students are unable to appreciate or consider the practical limitations that influence instrument design and data acquisition, and therefore, never question the significance or quality of the data. This multiple dilemma led the analytical program to focus on developing rugged, low-cost, low-maintenance, and low-power Small, Mobile Instruments for Laboratory Enhancement (SMILE), that could be easily constructed by students and so allowing students a peek inside the “black box”.
The basic premise of the proposal is that ‘constructing, tuning, and using instruments’, is an invaluable aid in understanding science and engineering concepts. A number of studies indicate that actual use of instruments impacts both students’ understanding of chemical concepts and their motivation and attitudes toward learning science. Our proposition has its roots in two key NRC reports. Science in the old school was taught by studying textbooks that reported the conclusions of what scientists know and have learned over time. Many of us were given opportunities to use the scientific method to perform hands-on experiments, with the focus being on how scientists know what they know. Much of this old-school science instruction is inconsistent with the principles highlighted in the NRC reports. Simply telling students what scientists have discovered, or asking students to follow the scientific method step-by-step, is not sufficient to change existing preconceptions about important scientific phenomena or to develop the knowledge, skills, and attitudes that will enable students to understand what it means to “do science” and participate effectively in a larger scientific community. These key reports suggest a number of guidelines: The knowledge-centered approach focuses on what is taught (subject matter), why it is taught (understanding), how it should be organized into a curriculum, and what competence or mastery looks like (learning goals, assessment). With these learning strategies and guidelines in mind, we have developed a novel method of teaching and engaging our students, a model we believe represents a very different approach to scientific inquiry, and highly worth disseminating to other institutions.
We have developed a co-curricular chemistry lab program where instruments, such as a colorimeter or fluorometer are built by the senior-level instrumental analysis students, and then used in lower-level general and analytical chemistry courses by their younger peers (and/or donated to local school districts). As the younger peers advance through the curriculum, their knowledge of the instrumentation and their ability to use the instrumentation in more sophisticated ways increases until, eventually, they are involved in the construction of a better version of the instrument to be used by their younger peers. The use of these student-built instruments promotes student competency in the sciences and engineering. We have found that it is a natural conduit for co-curricular experiences, and strongly fosters student ownership of their program's curriculum. The additional benefit of such instruments is that the low-price, low- maintenance and low-operating costs allows for deployment of multiple units. Some of the new instruments are donated to high schools for use in science class, thus allowing Penn State University to build connections with pre-college teachers. Our initial data suggests a significant increase in student engagement, interest, and understanding of related concepts in science.
Our overall scholarly interest focuses on better ways of teaching chemistry, building & utilizing instruments to positively impact student understanding. A literature search in this area reveals that very little work has been done on how students learn from instruments. What is widely reported is that use of multiple media such as books, television, and computers is useful, and that each type of media has unique capabilities that can influence student learning, but the advantages are very much dependent on the environment in which the student-media interactions take place. It is also reported that some students would learn regardless of the type of medium used to present the material. However, all students benefit from the unique capabilities of the medium if teaching strategies also took advantage of these capabilities. How this ‘multiple media’ is incorporated and brought together in a single instructional environment and strategically used to facilitate learning, matters greatly. Just like different forms of media, the understanding and use of scientific instruments, possess unique characteristics that could aid students in developing their understanding of chemistry concepts. Therefore, as part of on-going instrument development, we will also investigate in depth through our assessments the impact of analytical instrumentation, and the particular method we have created, on student learning of chemistry concepts.
Technology education is not the same as educational technology (also known as instructional technology). Educational technology involves using technological developments such as computers, audio visual equipment and mass media as tools to enhance and optimize the teaching and learning environment in all school subjects including technology education. Technology education is a school subject specifically designed to help students develop technological literacy. Technological literacy is defined as the ability to use, manage, assess and understand technology. Several reports stress the need for students to be engaged in activities that promote technological literacy through the development of knowledge and abilities necessary to make informed decisions regarding the use and management of technology. The study of technology enhances student learning by highlighting the relationships among technologies and between technology and other subjects. The goal of technological literacy is to provide people with the tools to participate intelligently and thoughtfully in the world around them.
Although students encounter instrumental techniques in courses other than analytical chemistry, instrumental analysis courses are the primary mechanism for providing technology education in the chemistry curriculum. It is within the instrumental analysis course where students get hands- on operating experience or detailed explanations of how instruments work.
In 2007, of the 12,925 students graduating with a degree in chemistry, only 4961 students (38%) continued into domestic graduate (M.S. and Ph.D) programs. It has been noted that industry and academic institutions often value different undergraduate experiences, skills and knowledge sets. Although the STEM industry ranks interpersonal and intrapersonal communication skills as important, when appropriately queried, most also cite technological competence to be as important. STEM related careers require persons able to competently operate instrumentation including knowing the components of instrumental systems, the ability to manage technology to ensure that all technological activities are efficient and appropriate, be able to make decisions about technology on an informed basis, and be able to understand and synthesize facts and information into new insights. Our own informal survey of 50 companies (28 respondents) indicates that an ability to modify, troubleshoot and maintain existing instrumentation is highly valued but a skill lacking in almost all undergraduates.
In contrast, many instrumental analysis (IA) courses survey 10 to 20 instrumental techniques within a typical one-semester (15-week) course. The laboratory component offers hands-on experience with a subset of these methods. Clearly, the existing structure may compromise the ability of even the best students to have a meaningful experience, i.e., to be able to obtain deep conceptual understanding of any one technique, due to information overload.
Unfortunately, this “National Model” for IA renders a senior-level capstone course in analytical chemistry to nothing more than a general survey course. In response, the analytical program at Penn State reduced the breadth of material (number of instruments discussed in lecture/lab) and developed a more rigorous in-depth comprehensive exposure for a select subset of instruments. Lecture provides the theory; problem sets and lecture activities reinforce those concepts; and the laboratory experience provides the practical application of the theory. Why this format?
The traditional course structure looks like this:
​
​
​
​
​
​
​
​
If each of the vertical boxes represents a core concept, then the traditional survey course provides a broad overview of many core concepts but at the expense of any in-depth exposure to any one core area. Beyond the course, a student wishing to learn more about any given area (for employment or academic purposes) must essentially start from scratch…the same as if they were approaching a new core concept not touched upon previously (the last vertical box in the diagram).
The current IA format in the analytical program looks like this:
Because a student has a more in-depth exposure to the material, it is easier to grasp more difficult concepts and facilitates understanding in new areas because of the richness of their exposure.
In 1997, the Alfred P. Sloan Foundation established a funding initiative to promote the development of Professional Science Masters (PSM) programs. The Foundation recognized that the existing path to a doctorate degree might not be the best for many science-trained professionals, given the long period of study required, the low rate of completion and the difficult labor market. The National Research Council subsequently endorsed the expansion of PSM programs because of the cognizant need in industry for employees who have deep scientific knowledge as well as skills to apply that knowledge in innovative ways. Despite the growth in the number of PSM programs, there is still considerable opportunity within the undergraduate chemistry program to better serve the needs of industry.
We are not advocating radical change in the manner in which instrumental analysis courses are taught. However, we do believe that the curriculum can be more responsive to industry needs as well as graduate programs in the chemical sciences. Truly, the growth of modern instrumental methods of analysis has paralleled the development of the electronics and computer industries. The SMILE approach, whether instituted as a single modular component or as a unifying theme to achieve integration of course topics, uses electronics and design layout as a platform for understanding chemical technology. All spectroscopic and chromatographic instrumental methods of analysis are interrelated on this level.
Nation-wide from 1981-1998, instrumental analysis courses underwent a sea-change in curriculum offerings, but more so in the laboratory than in the lecture. One study found that out of a choice of thirty-seven potential instrumental topics, only six topics received more teaching time (lecture and lab) in 1998 than in 1981. The percentage of instructional time spent on electronics in instrumental analysis courses increased by 10%. The increase in instructional time spent on electronics likely reflects the rise in sophistication of the analytical tools at our disposal, which requires a higher level of understanding to manipulate and troubleshoot instrumentation, and an increase in cost of chemical instrumentation. In contrast, many of our peers report that student interest in electronics is low and this attitude has an overwhelming influence (in a negative way) on course evaluations. This was certainly the case here at Penn State.
In order to increase student interest in electronics and promote an appreciation for the practical applications of electronics in chemistry, electronics-based projects were introduced in the instrumental analysis course eleven years ago. SMILE requires students to build, from scratch, calibrated and quantitative instruments (e.g., colorimeter, fluorometer, a diamond-anvil cell, gated-fluorometer, barcode scanner, GC-FID, ion chromatography instrument, dissolved oxygen probe, cyclic voltammetry instrument, DNA oligomer sensor, UV-VIS grating-based photodiode array spectrometer, CE-chip, among others). The semester-long projects provide an intensive guided-inquiry learning experience. Students are the principal investigators; they must submit a literature-based research proposal, work as a team to complete the project, present their research at a chemistry-wide poster symposium, and write a final report. Faculty guidance does not ensure a successful end to any project. The success or failure of any project is based on the students’ initiative, motivation, organization and work ethic.
An important question is whether SMILE enhances student comprehension of chemical concepts. One method of evaluation measures student satisfaction of the learning experience and perceived outcomes. Introducing the projects into the curriculum was a substantial risk because 75% of the course grade is attributed to electronics-based topics – a course topic students clearly identified as their least favorite topic (100% of student respondents on course evaluations, 2001-2003). Instead, the projects became a positive life-defining experience for many of our students. Over the past 20 years, 80% of the students consistently indicate that electronics and the electronic projects are their favorite topics/activities in the course.
“Often times students are given projects that, on the surface appear to be simple and straightforward but actually have much deeper intentions. Such a project was given to us the last semester of our senior years at Penn State…At first glance, this project seemed a little intimidating…In our eyes the project was a success not because we completed the project, but because we accomplished a task we never thought we could. In retrospect, we learned to start small and build piece-by piece. We achieved the ability to question why things work the way they do and how to network and use all available resources...We were able to take an overwhelming project and we stepped up to its challenges. By taking each process step- by-step, by networking, by not being afraid of trying new things, and by accepting, at first, frustration and then working at finding a solution to the problem success was achieved.”
“…Second I wanted to thank you for the opportunities you gave me in your chromatography and instrumentation classes. I know you said at the time that what we were learning was important and would give us an edge in the real world but I didn't believe you. My new opinion is that the experience I had in those two classes, more than anything else, helped me get this job. Not only get the job but be competent in it as well. Third I wanted to see if I could get some help from you in the future if I needed it. We just got a brand new Dionex ICS-3000 system and I am the only person in the building that knows how to use it. When the Dionex guy came to set it up he was quizzing us on the instrument and I was the only person who knew what an offset voltage was. I thought you'd appreciate that…”.
However, student self-assessment alone is not a sufficient indicator of learning outcomes. For two consecutive semesters (2009-2010), an informal assessment of the SMILE program within the instrumental analysis spectroscopy course has been conducted. Typical enrollment within the course is 30 students per semester with 10 students in each of three four-hour laboratory sections. In each semester, SMILE was incorporated into one laboratory section whereas the other two sections were used as control groups. In the SMILE and control laboratory sections, students were divided into five groups of two students each. During a two week modular set of exercises, the SMILE groups built either a colorimeter or a fluorometer. Although the instruments were built from kits, during the first week of the module each group worked with the instructor to develop a circuit diagram and design layout for a fluorometer/spectrophotometer. The exercise reinforced prior lecture and laboratory activities on electronics and spectrometer design and required the students to “synthesize” the information in the development of a basic circuit design for fluorescence/absorption measurements. Once completed, students compared their “custom circuit and instrument design” to that of the SMILE instrument kit. The close correspondence between their proposed design and that used in the kit provided a sense of accomplishment and competency. In the second week, each group built their assigned instrument and began initial testing and validation. Each group was required to develop three laboratory activities using their instrument but received no additional in-class time in which to complete the project.
The control groups conducted a two-week study which incorporated fluorescence, UV-VIS absorption and IR/Raman spectroscopy using research-grade instrumentation (Fluorolog 3, Cary 4000, Nicolet 6700). In the first exercise, students investigated excimer and non-excimer formation in pyrene and anthracene solutions, respectively. Students collected the absorption spectra to obtain the appropriate excitation wavelengths then obtained the fluorescence emission profiles for pyrene and anthracene as a function of concentration. Next students investigated the inner filter effect using synchronous scanning fluorescence (SFS) on a mixture of four polynuclear aromatic hydrocarbons (fluorene, anthracene, perylene, benzofluorene). SFS is a useful technique for isolating the fluorescent signals of each component in a simple mixture for quantitation purposes without requiring a prior chromatographic step. Finally, students collected the emission spectra of water at five different excitation wavelengths then calculated the corresponding IR/Raman vibrational mode and compared/plotted the calculated frequencies on an IR spectrum of water.
The assessment compared the answers between the SMILE and control groups on two sets of questions:
-
Two short essay questions on one of the midterm exams
-
On the attached datasheets are the specifications for three LED sources and three silicon photodiodes. Choosing one of each, design a simple circuit that will power the LED and collect and process (including A-to-D conversion) the signal from the photodiode at the λmax of your LED. Include the nominal values of all components and the signal voltage output range to the converter. Provide a basic block diagram layout of your spectrophotometer.
-
Explain why there is such a difference in sensitivity between fluorescence and absorption instrumentation.
-
-
A set of in-class questions – for one lecture period each week students work in small groups (3 to 4 students) to discuss a set of in-class questions (termed a Lecture Activity). Although students are permitted to self-assemble into small groups, each student is required to submit their own answers prior to leaving class.​
The final question in the lecture activity was repeated on the later midterm exam. The purpose was to determine if scores would improve on a question students previously considered and had received feedback on their responses. Students in the SMILE cohort, on average, scored 84%
and 87% on the two midterm questions compared to 81% and 81% for students in the control group. Given the small numbers of students (30 each semester for a total of 60 students), the differences may not be statistically significant. In contrast, students in the SMILE cohort scored, on average, 90% on the Lecture Activity compared to an average score of 78% in the control groups. The difference in performance is striking but may diminish with a larger dataset.
Overt he past 20 years, we have partnered with a number of institutions and organizations to implement a SMILE experience or instrument within their curricula:
-
Park Forest Middle School, PA
-
West Branch Middle School, PA
-
Southern Huntingdon High School, PA
-
Solroed Gymnasium, Solroed Strand, Denmark
-
Department of Chemistry, Rollins College
-
Chemical Sciences Program, Mohawk College, Hamilton, Ontario, Canada
-
Math and Natural Sciences Department, Centenary College
-
Department of Chemistry, Campbellsville University
-
Department of Chemistry, Indiana University
-
Department of Chemistry, Concordia College
-
Department of Chemistry, SUNY Buffalo
-
Saraya Biochemical Laboratory, Osaka, Japan
-
Bioengineering/Mechanical Engineering, Georgia Institute of Technology
-
Department of Chemistry, Simon Fraser University
-
Department of Chemistry, Assam University, India
-
Universidade Federal do Parana, Brazil
-
Department of Computer Engineering, Mapua University, Philippines
-
Analytical Chemistry courses at the Penn State UP campus
-
General chemistry laboratories at the Penn State Harrisburg and UP campuses
-
Loveland Living Planet Aquarium, Utah
-
National Oceanic and Atmospheric Administration Fisheries
-
Center for Science and the Schools (CSATS), ReBuild Program, Penn State/Government of Ghana collaboration
-
Environmental Science Centre, British Geological Survey
-
Department of Food Science and Technology, Oregon State University

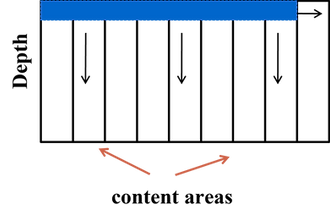
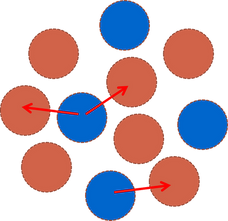